Project
A look at goals, methods and measurement devices of the CLOUDLAB project.
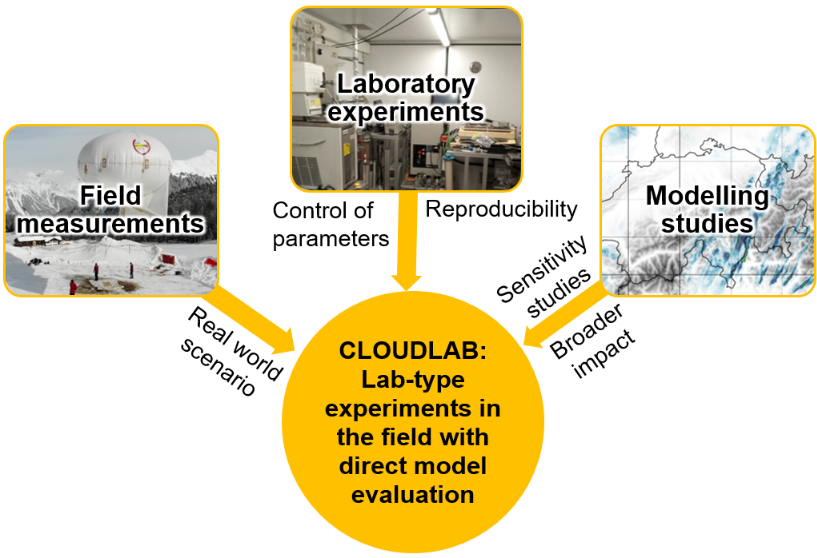
CLOUDLAB
The ETH project CLOUDLAB aims at advancing the understanding of cloud physics by performing targeted seeding experiments to study cloud processes and precipitation formation using a multi-dimensional set of observations and numerical modelling. The gained knowledge will help to improve weather forecasts and climate projections.
Challenges and approach
Clouds are an atmospheric phenomenon central to the hydrological cycle and the Earth’s climate. Most of the precipitation originates from clouds containing ice. However, major gaps still exist in our knowledge of microphysical processes, especially associated with the formation and growth of ice. This emphasizes the need to advance our understanding of these processes with the help of innovative cloud observation techniques.
CLOUDLAB utilizes wintertime stratus clouds as a natural laboratory to perform targeted seeding experiments (i.e., controlled perturbations) in a stable environment to study cloud processes and precipitation formation. Due to the persistence of stratus clouds, this approach allows repeating the seeding experiments under similar and realistic initial conditions and thus bridges the gap between laboratory and field observations. One seeding experiment involves two steps: In the first step, ice nucleating particles (i.e. aerosol particles that aid ice formation) are injected by a drone into supercooled stratus clouds to induce ice crystal formation and subsequent growth processes. In the second step, the related microphysical changes are measured by extensive instrumentation, including ground-based remote sensing instruments (e.g. cloud radar), a tethered balloon system, and an additional drone. The repetitive introduction of local perturbations in a stable environment allows inferring the ice formation and growth rates, directly furthering our understanding of precipitation initiation.
The analysis of the cloud seeding events will be used to validate and improve the cloud microphysics scheme in the weather forecast model ICON (i.e., ICOsahedral Non-hydrostatic model; will be operationally used by MeteoSwiss) and ultimately increase precipitation forecast skills. In addition, the gained knowledge during CLOUDLAB will be important to quantify the consequences of artificial weather modification and climate interventions.
Instrumentation
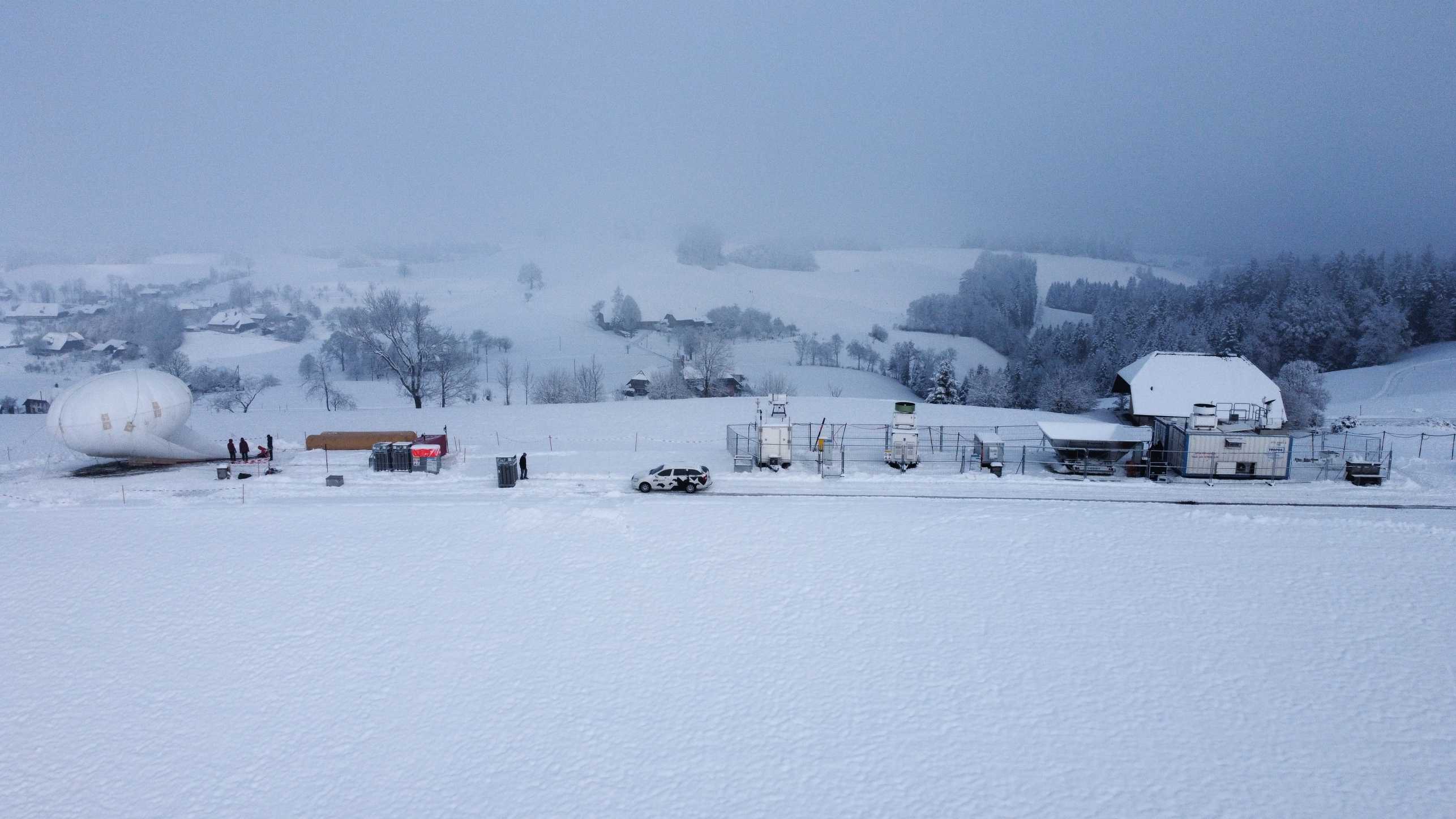
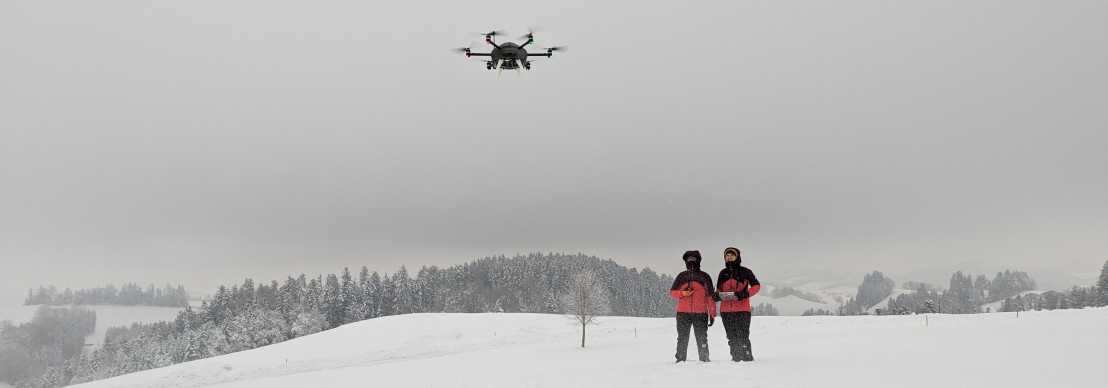
Our seeding UAV from external page Meteomatics is arguably the most important player in CLOUDLAB seeding experiments. We attach the seeding flares from external page Cloud Seeding Technologies to the bottom of the UAV, fly the UAV to precisely where in the cloud we want to seed (based on wind direction, wind speed, temperature, and cloud structure), and then the flares burn while we fly our desired seeding pattern. The flares contain silver iodide, which are very efficient ice nucleating particles. Thus, we can initiate the formation and growth of ice crystals which we later measure downwind with the instrumentation at Rapier Platz.
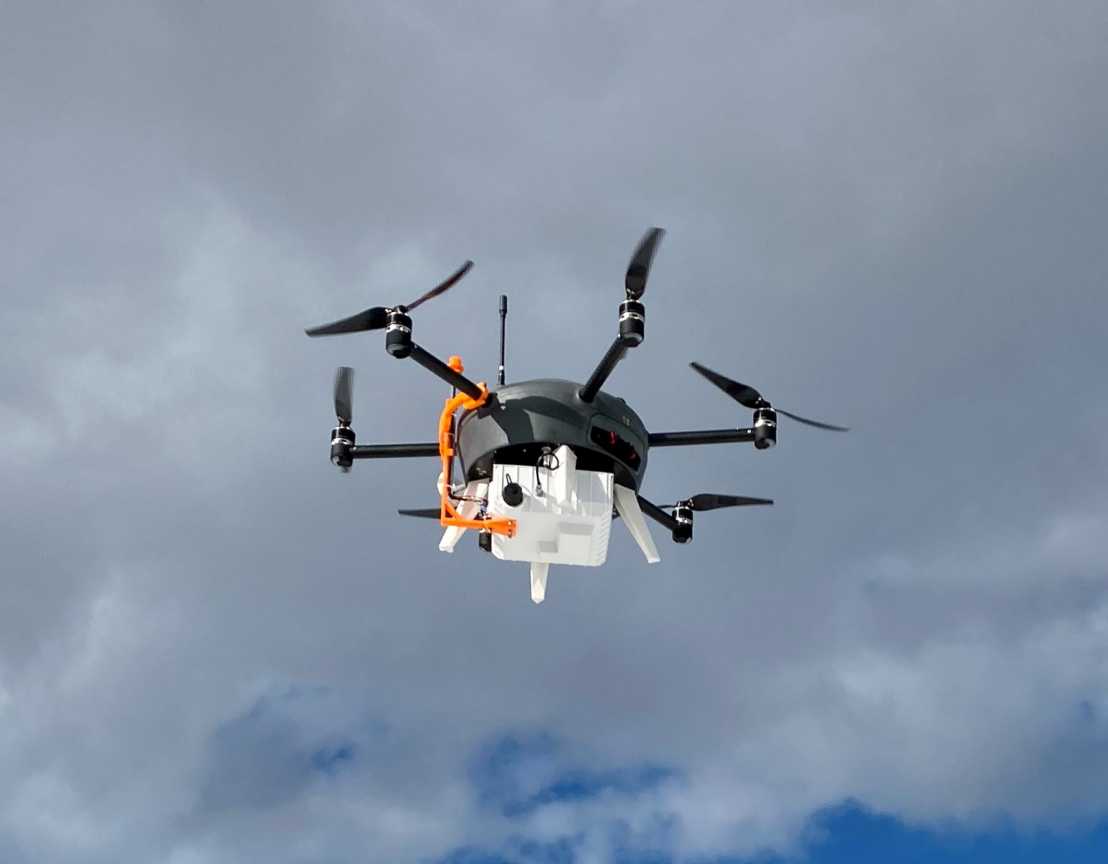
We have two Portable Optical Particle Counters (POPS), which are small devices that measure aerosol size and concentration in the range of 120 nm to 3 micrometers.
One POPS is mounted on a drone, and we can use that to measure aerosol at many different locations and altitudes. In cloud seeding experiments, we can use the POPS-drone to fly through the seeding plume and measure the distribution of seeding particles in the air.
The second POPS is mounted on the rack of the tethered balloon system (TBS, also called Holoballoon). This POPS is useful during the cloud seeding experiments because it tells us in real-time when the seeding plume is passing by the balloon.
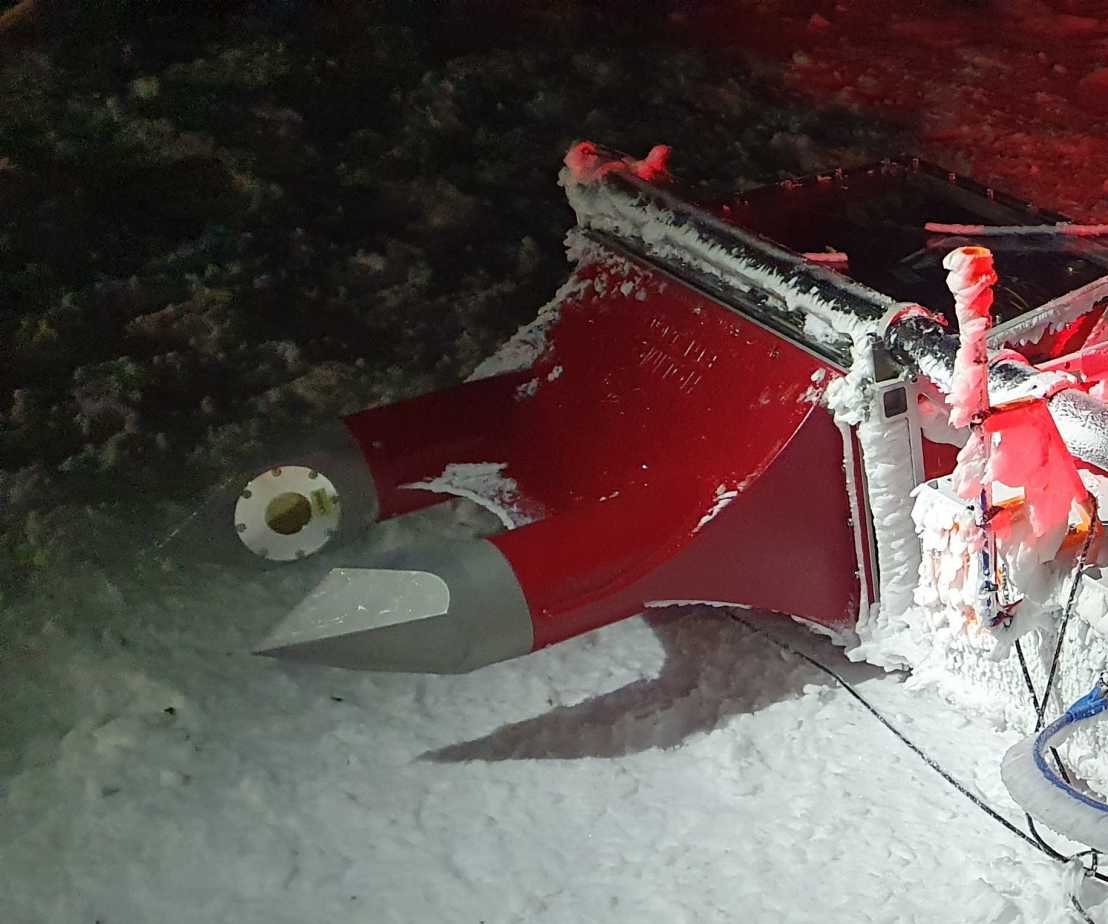
We developed the field instrument HOLIMO (HOLographic Imager for Microscopic Objects) which uses digital in-line holography in open path configuration to in-situ image ensembles of cloud particles within a well-defined sample volume (i.e. a sample volume independent of particle size and wind speed). Two-dimensional images of single cloud particles between 6 µm and up to several millimeters are algorithmically extracted. Particle shape is analyzed to classify cloud particles larger than 25 µm as water droplets (circular) or ice crystals (noncircular). Thus, phase- and spatially-resolved size distributions, concentrations, and cloud water contents are obtained.
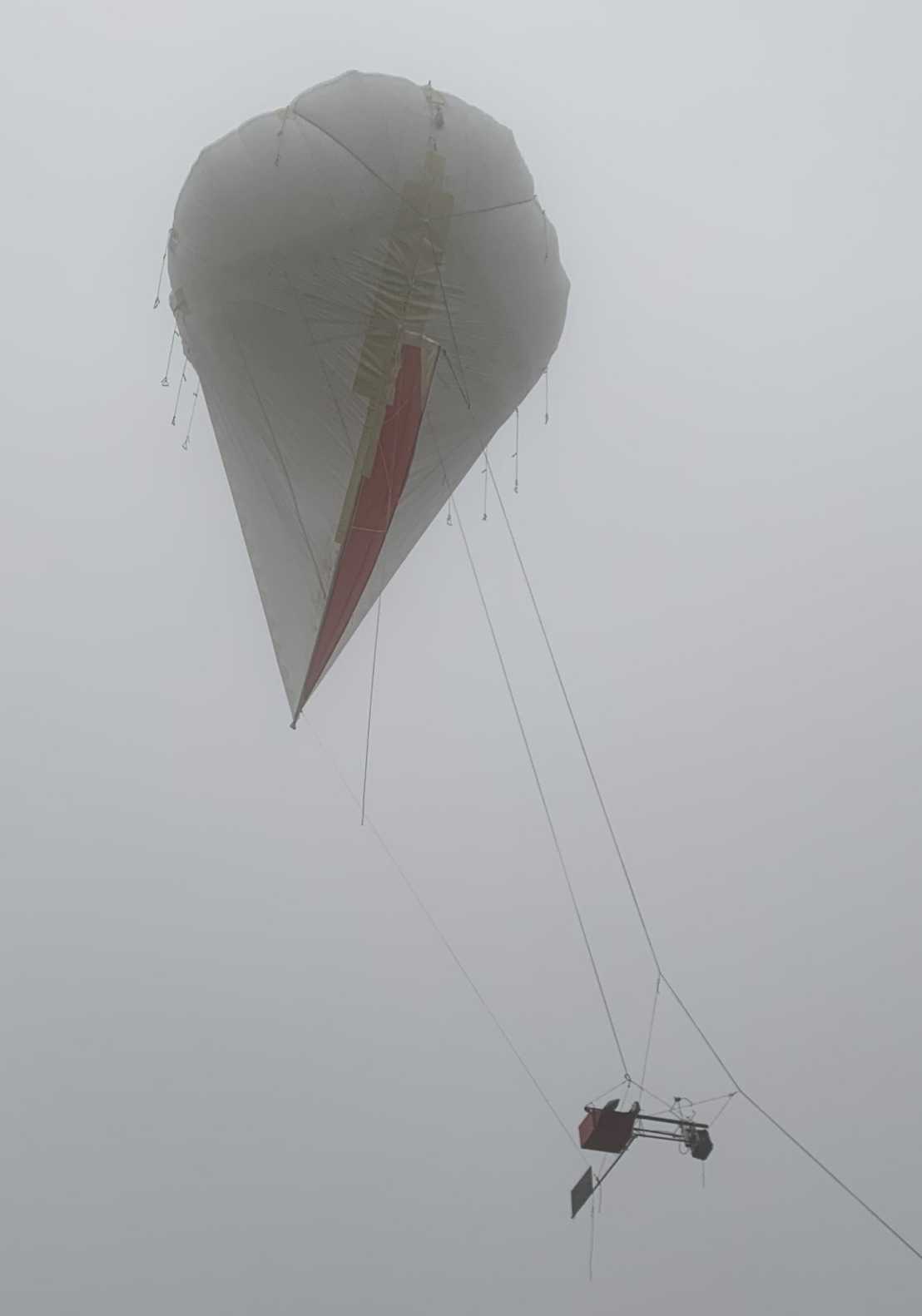
HoloBalloon, our newest measurement platform, is the combination of HOLIMO and a tethered balloon system (200m3). HoloBalloon can fly up to a height of 1000 m above ground level and is retreived with a winch powered by a V8 Chevy engine. HoloBalloon is also equipped with auxiliary instruments to observe temperature, relative humidity and GPS position. Similar to our measurement platform HoloGondel, we can obtain vertical profiles with HoloBalloon with a high spatial resolution due to the relatively low wind speed.
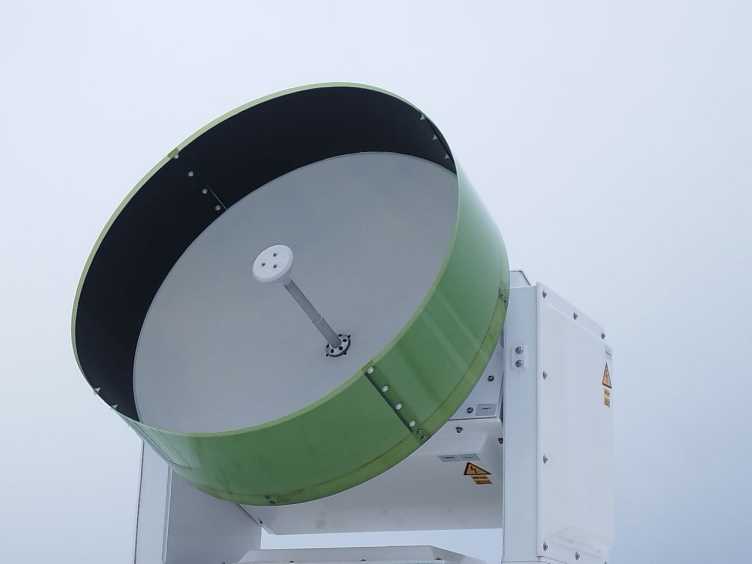
Within CLOUDLAB we operate several cloud radars (millimeter wavelength) in scanning and/or vertical mode (see visualisation) to understand when good seeding conditions prevail, namely if a cloud is present on site, how thick it is, what the background reflectivity is and then see the microphysical changes induces by seeding. For this purpose we are also able to "scan" with the radars by varying azimuth and/or elevation. To ensure application of typical retrievals, one radar was always kept vertical while we could scan with up to two radars at our main site thanks to our partners from external page TROPOS.
Usually scans are done either as Plan Position Indicator (PPI, varyiing azimuth at fixed elevation) or as Range Height Indicator (RHI, varying elevation at fixed azimuth) to get the spatial pattern of clouds or to get the wind field. For our seeding experiments with a flare that burns for roughly six minutes we run such scans only partially but repeatedly, i.e. either a limited azimuth range at a fixed elevation (partialPPI / sector scan) or at a fixed azimuth and varying the elevation (elevation scan). You can find the visualized scans on our quicklook website. Generally, we repeat the scans for around 20 minutes to get an idea about the pre-seeding background, get the seeding signal, and the background afterwards. With a scan speed of 1° per second and a scan range of around 90° a scan takes 90 seconds, or in other words the six minutes burn time is covered by 4 scans (or more when we run a smaller scan range).
When we are not running seeding experiments we still record data, and thanks to the different frequency, we are also able to conduct performance comparisons between the radar. The wavelengths and number of radars that we operate are as follows:
- 2x scanning Ka band radar (Metek Mira, 35 GHz frequency/8 mm wavelength), during winter 2022 only one of them was available
- 1x vertical pointing and 1x scanning W band radars (RPG FMCW, 94 GHz frequency/3 mm wavelength), where the scanning radar was only available for winter 2023/2024
- 1x scanning X band radar (Prosensing SSXPOL, 9 GHz frequency/33 mm wavelength) , only available for winter 2023/2024
On site we have installed a single wavelength (940 nm) lidar, often also called simply ceilometer, to detect cloud base (and planetary boundary layer based on aerosol concenctrations) as well. This CHM15k ceilometer from Lufft runs continuously at 15 seconds sampling time, 24/7/365 with minimal maintenance. and supplements the cloud radar measurements and delivers information about how the liquid water droplets in the cloud and when cloud base is too low for the cloud radars helps to detect it.
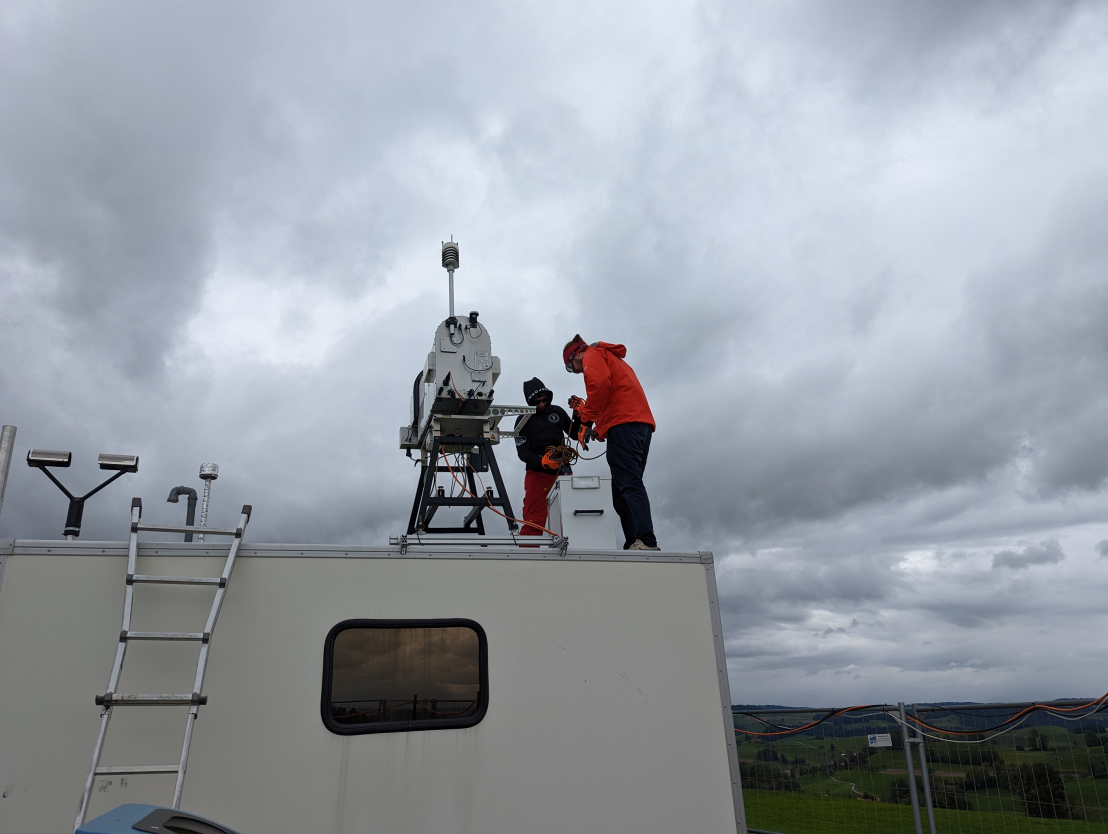
A microwave radiometer is capable to derive a vertical temperature (and humidity) profile from seven channels of different wavelength. This allows us to get a liquid water path (the amount of water in the whole column of air) as well. This in turn can be used to derive liquid water content of the cloud.
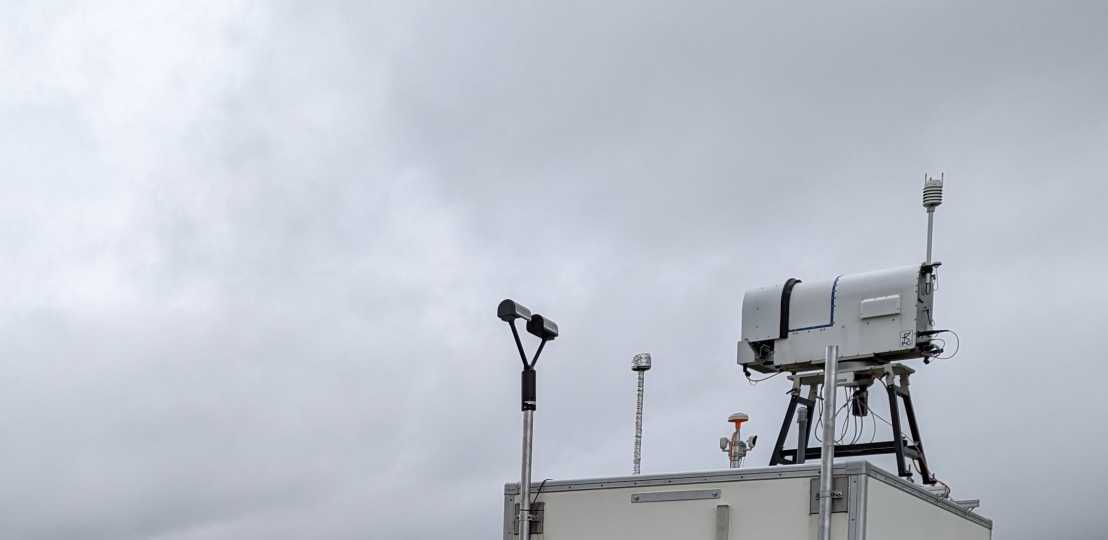
Our disdrometer is a external page parsivel from Ott hydromet which measures droplets falling through a laser path and derives fall speed and size from its speed and blockage of the laser. Based on this a rain rate which corresponds to a radar reflectivity and can be used as ground truth for cloud radar calibration.
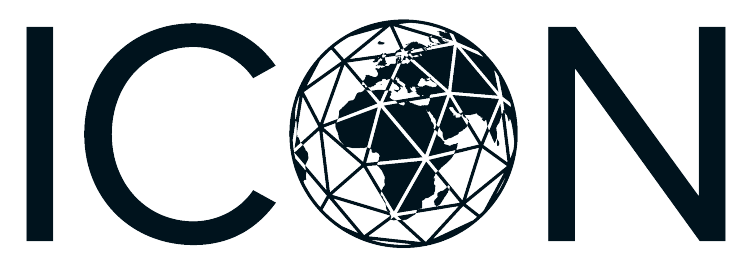
We employ the numerical weather model ICON (external page https://gitlab.dkrz.de/icon/icon-model) that is co-developed by DWD, MPI-M, MeteoSwiss, and ETH. The unique grid structure allows for flexible adaptations to horizontal resolutions compared to traditional rectangular weather models. Within CLOUDLAB, we require a highly granular grid with resolutions down to 65 m to simulate the conducted seeding experiments in the model. We also introduced a more recent parameterization (Marcolli et al., 2016) for the seeding particles to initiate the ice phase inside the simulated cloud. The unique data set from the field campaigns allows for a unprecedented model-observation comparison and sheds light on misrepresentations in the model.